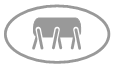 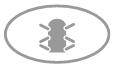 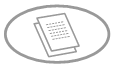 
Motivation
The methodology of locomotion has
undergone dramatic change with the advancement of human civilization. During
the last two centuries, various remarkable modern transportation methods,
including wheeled and track vehicles, airplanes, were invented based on the
discovered physical principles and designed to adapt to a specific
environment. However, development of vehicles that are capable of passage
over natural rough terrains remains primitive. Are humans sufficiently
clever to invent a new machine to serve this purpose using presently known
design theories and methodologies? Perhaps, but I am more inclined to
believe that a systematic and incremental approach building on learning the
lessons from nature offers a more likely path.
Animals provide an ideal
inspiration to the legged robotic world. After millions of years of
evolution, animals have developed high complex degree-of-freedom (DOF)
systems yet perform great mobility that is unparallel to the existing
robotic machines. Though animals’ appearances and structures may vary
significantly, for similar behaviors, for example, running, they seem to
share a few similar fundamental motion patterns which can be represented by
simple low DOF spring-damper-mass models (“templates”). Recent-developed
bio-inspired legged machines such as RHex and iSprawl whose running motion
can be represented in the similar model as in animals indeed have begun to
exhibit a much greater mobility. Of course nature has its own mechanism for
evolution and formats animals into high DOF systems after
generations---reflecting most likely the necessity of performing a wide
diversity of behaviors to ensure survival? Probably true. No matter what the
answer is, this concept opens a new challenge yet fundamental question: How
to systematically design and control a robot (or a general physical dynamic
system) based on a bio-inspired model or even multi-models? I believe (1)
systematically learning from the biological design and behavior offers solid
concept and intuition of dynamic locomotion. (2) Analyzing a particular
behavior by assigning it a distinguished, simple model opens the gateway to
truly understand the essence of design and control of each particular
constituent of the larger behavioral suite. (3) With right composition of
multi templates where each presents a unique behavior, this approach to
research promises the capability in the long run to design and control a
much more complex high-mobility system capable of performing multi agile
behaviors and responding to diverse nature environments.
I have no doubt that someday the
legged machines will become one of human’s major transportation methods as
“car” was invented as wheeled human-carrier 100 years ago. However, it is a
challenge task even for a small-size robust and agile legged machine to
achieve the modest capabilities that would entitle use to call it a “robot”.
Research Work
My research activities and
interests lie in the understanding/improvement of performance/behavior of
bio-inspired legged machines (short-term) and general physical dynamic
systems (long-term) from two aspects: design and control. Design with
the appropriate morphology and materials (“form”) forms the substrate on
which system performance can be elicited by appropriately matched control
(“function”). In my graduate and post-doctoral research I have worked with
diverse teams on various portions, detailed in the summaries listed below,
of this long-term task as depicted in this figure:
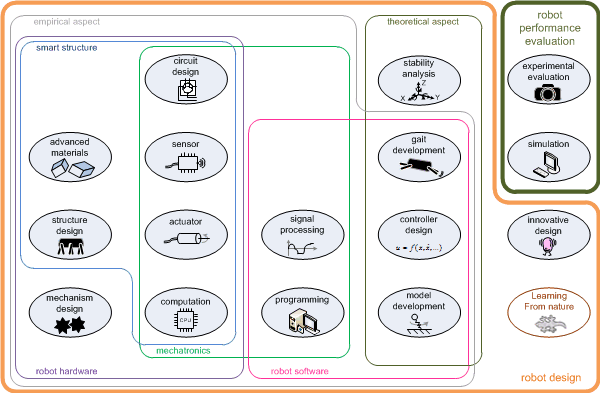
|
Design of a Bio-inspired Dynamical Vertical Climbing Robot |
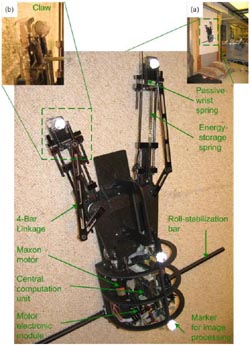
Movies:
Front view
Side view |
 This
paper reviews a template for dynamical climbing originating in biology,
explores its hypothetical utility , and offers a preliminary look at
empirical data bearing on the feasibility of adapting it to build a
robot that “runs” vertically upward. The recently proposed pendulous
climbing model abstracts remarkable similarities in dynamic wall scaling
behavior exhibited by radically different animal species. The present
paper’s first contribution summarizes a continuing numerical study of
this model to hypothesize that these animals’ apparently “wasteful”
commitments to lateral oscillations may be justified by a significant
gain in the dynamical stability and, hence, the robustness of their
resulting climbing capability. We explore numerically a scaled version
of this template devised to inform the design of a physically realizable
robotic mechanism with the same climbing behavior. The paper’s second
contribution documents the design and offers very preliminary empirical
data arising from a physical instantiation of this model.
Notwithstanding the significant differences between the proposed
bio-inspired template and its physical robot model, these intial data
suggest the mechanical climber may be capable of roughly reproducing
both the motions and ground reaction forces characteristic of dynamical
climbing animals. Even without proper tuning the robot’s steady state
trajectories manifest a substantial exchange of kinetic and potential
energy, resulting in vertical speeds of 0.14 m/s (0.35 bl/s) and
claiming its place as the first bio-inspired dynamical legged climbing
platform.
(in collaboration with Dr. Jonathan Clark) |
|
Spontaneous formation of 1D ripples in transit to highly-ordered 2D
herringbone structures through sequential and unequal 2D mechanical
force |
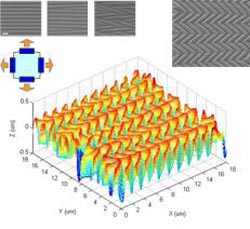 |
Spontaneous
formation of periodic structures with controlled morphologies on
surfaces has been of great interest for many potential applications. We
report the formation of various submicron wrinkle patterns using
mechanical force coupled with oxygen plasma treatment on PDMS. It allows
us to control the amount and timing of strain applied to the substrate
on both planar directions (either simultaneously or sequentially), which
appears to be critical to maneuver the pattern formation of 1D ripple,
2D herringbone, and patterns in between in real time. We observe clear
transitions from ripple, to ripple with bifurcation, to
ripple/herringbone mixed features, and to completely 2D herringbone
structure. More specifically, we demonstrate the well-controlled
formation of a highly-ordered zigzag-based herringbone structure, and
elucidate the mechanisms of pattern formation and transition at a large
strain level (up to 60%). |
|
Distributed Mechanical Feedback in Arthropods and Robots Simplifies
Control of Rapid Running on Challenging Terrain |
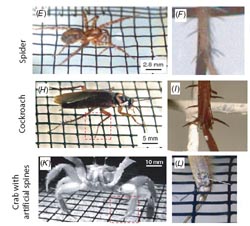 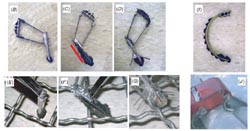
some movies |
 Terrestrial
arthropods negotiate demanding terrain more effectively than any
search-and-rescue robot. Slow, precise stepping using distributed neural
feedback is one strategy for dealing with challenging terrain.
Alternatively, arthropods could simplify control on demanding surfaces
by rapid running that uses kinetic energy to bridge gaps between
footholds. We demonstrate that this is achieved using distributed
mechanical feedback, resulting from passive contacts along legs
positioned by pre-programmed trajectories favorable to their attachment
mechanisms. We used wire-mesh experimental surfaces to determine how a
decrease in foothold probability affects speed and stability. Spiders
and insects attained high running speeds on simulated terrain with 90%
of the surface contact area removed. Cockroaches maintained high speeds
even with their tarsi ablated, by generating horizontally oriented leg
trajectories. Spiders with more vertically directed leg placement used
leg spines, which resulted in more effective distributed contact by
interlocking with asperities during leg extension, but collapsing during
flexion, preventing entanglement. Ghost crabs, which naturally lack leg
spines, showed increased mobility on wire mesh after the addition of
artificial, collapsible spines. A bioinspired robot, RHex, was
redesigned to maximize effective distributed leg contact, by changing
leg orientation and adding directional spines. These changes improved RHex’s agility on challenging surfaces without adding sensors or
changing the control system.
(in collaboration with Dr. J. C. Spagna, Prof. D.
I. Goldman, and Prof. R. J. Full at UC - Berkeley) |
|
RHex-SLIP: A Model of the Robotic Hexapod RHex in the Sagittal Plane |
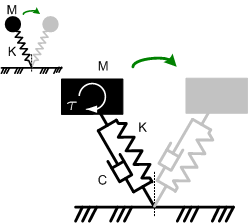 |
 The
spring-loaded inverted pendulum (SLIP) is a simple, passively-elastic
two-degree-of-freedom model for legged locomotion that describes the
saggital-plane center of mass (COM) dynamics of many animal species and
some legged robots. In previous work we have extended SLIP to model
three-dimensional COM motions and to incorporate multiple stance legs.
To better understand the agile hexapedal robot RHex, here we incorporate
key details of leg design and motor specifications into SLIP, allowing
us to match SLIP gaits with experimental data from RHex, and to
investigate their stability properties. We find that motor and leg
characteristics, and leg touchdown and liftoff protocols, can
significantly influence stability, and that non-periodic "chaotic" gaits
can occur.
(in collaboration with Dr.
J. Seipel and Prof. P. Holmes at Princeton University) |
|
Sensor
Data Fusion for Body State Estimation in a Hexapod Robot with Dynamical
Gaits |
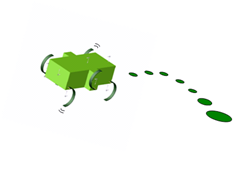  |
We
report on a hybrid 12 dimensional full body state estimator for a
hexapod robot executing a jogging gait in steady state on level terrain
with regularly alternating ground contact and aerial phases of motion.
We use a repeating sequence of continuous time dynamical models that are
switched in and out of an Extended Kalman Filter to fuse measurements
from a novel leg pose sensor and inertial sensors. We implement this
estimation procedure offline, using data extracted from numerous
repeated runs of the hexapod robot RHex (bearing the appropriate sensor
suite) and evaluate its performance with reference to a visual ground
truth measurement system, comparing as well, the relative performance of
different fusion approaches implemented via different model sequences. |
|
Advanced Inertia Measurement Unit (IMU) --- with 12-Axis Accelerometer Suite |
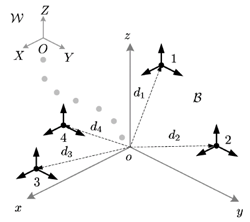 |
This
inertial measurement unit supplements the traditionally paired
three-axis rate gyro and three-axis accelerometer with a set of three
additional three-axis accelerometer suites, thereby providing additional
angular acceleration measurement, avoiding the need for localization of
the accelerometer at the center of mass on the robot's body, and
simplifying installation and calibration. |
|
Legged
Odometry from Body Pose in a Hexapod Robot |
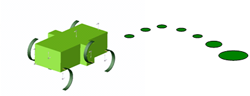 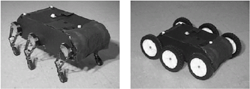 |
We
report on a continuous time odometry scheme for a walking hexapod robot
built upon a previously developed leg-strain based body pose estimator.
We implement this estimation procedure and odometry scheme on the robot
RHex and evaluate its performance at widely varying speeds and over
different ground conditions by means of a 6 degree of freedom vision
based ground truth measurement system (GTMS). We also compare the
performance to that of sensorless odometry schemes --- both legged as
well as on a wheeled version of the robot --- using GTMS measurements of
elapsed distance. |
|
A Leg
Configuration Sensory System for Dynamical Body Pose Estimation |
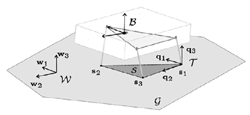 |
We
report on a novel leg strain sensory system for the autonomous robot
RHex implemented upon a cheap, high performance local wireless network.
We introduce a model for RHex's 4-bar legs relating leg strain to leg
kinematic configuration in the body coordinate frame. We compare against
ground truth measurement the performance of the model operating on
real-time leg strain data generated under completely realistic operating
conditions. We introduce an algorithm for computing six degree of
freedom body posture measurements in world frame coordinates from the
outputs of the six leg configuration models, together with a priori
information about the ground. We discuss the manner in which such stance
phase configuration estimates will be fused with other sensory data to
develop the continuous time full body state estimates for RHex. |
|
A
Context-Based State Estimation Technique for Hybrid Systems |
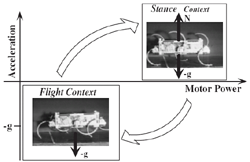 |
This
paper proposes an approach to robust state estimation for mobile robots
with intermittent dynamics. The approach consists of identifying the
robot’s mode of operation by classifying the output of onboard sensors
into mode-specific contexts. The underlying technique seeks to
efficiently use available sensor information to enable accurate,
high-bandwidth mode identification. Context classification is combined
with multiple-model filtering in order to significantly improve the
accuracy of state estimates for hybrid systems. This approach is
validated in simulation and shown experimentally to produce accurate
estimates on a jogging robot using low-cost sensors.
(in collaboration with Dr. S. Skaff, Prof. A. Rizzi, and Prof. H. Choset at Carnegie
Mellon University) |
|
|
|
|
|
|
|
|